- Forklift Lithium Battery
-
48V
- 48V 210Ah
- 48V 300Ah
- 48V 420Ah (949 x 349 x 569 mm)
- 48V 420Ah (950 x 421 x 450 mm)
- 48V 456Ah
- 48V 460Ah (830 x 630 x 590 mm)
- 48V 460Ah (950 x 421 x 450 mm)
- 48V 460Ah (800 x 630 x 600 mm)
- 48V 460Ah (820 x 660 x 470 mm)
- 48V 500Ah
- 48V 560Ah (810 x 630 x 600 mm)
- 48V 560Ah (950 x 592 x 450 mm)
- 48V 600Ah
- 48V 630Ah
-
48V
- Lithium Golf Cart Battery
- 12V Lithium Battery
12V 150Ah Lithium RV Battery
Bluetooth App | BCI Group 31
LiFePO4 Lithium
Discharge Temperature -20°C ~ 65°C
Fast Charger 14.6V 50A
Solar MPPT Charging - 24V Lithium Battery
- 36V Lithium Battery
- 48V Lithium Battery
-
48V LiFePO4 Battery
- 48V 50Ah
- 48V 50Ah (for Golf Carts)
- 48V 60Ah (8D)
- 48V 100Ah (8D)
- 48V 100Ah
- 48V 100Ah (Discharge 100A for Golf Carts)
- 48V 100Ah (Discharge 150A for Golf Carts)
- 48V 100Ah (Discharge 200A for Golf Carts)
- 48V 150Ah (for Golf Carts)
- 48V 160Ah (Discharge 100A for Golf Carts)
- 48V 160Ah (Discharge 160A for Golf Carts)
-
48V LiFePO4 Battery
- 60V Lithium Battery
-
60V LiFePO4 Battery
- 60V 20Ah
- 60V 30Ah
- 60V 50Ah
- 60V 50Ah (Small Size / Side Terminal)
- 60V 100Ah (for Electric Motocycle, Electric Scooter, LSV, AGV)
- 60V 100Ah (for Forklift, AGV, Electric Scooter, Sweeper)
- 60V 150Ah (E-Motocycle / E-Scooter / E-Tricycle / Tour LSV)
- 60V 200Ah (for Forklift, AGV, Electric Scooter, Sweeper)
-
60V LiFePO4 Battery
- 72V~96V Lithium Battery
- Rack-mounted Lithium Battery
- E-Bike Battery
- All-in-One Home-ESS
- Wall-mount Battery ESS
-
Home-ESS Lithium Battery PowerWall
- 24V 100Ah 2.4kWh PW24100-S PowerWall
- 48V 50Ah 2.4kWh PW4850-S PowerWall
- 48V 50Ah 2.56kWh PW5150-S PowerWall
- 48V 100Ah 5.12kWh PW51100-F PowerWall (IP65)
- 48V 100Ah 5.12kWh PW51100-S PowerWall
- 48V 100Ah 5.12kWh PW51100-H PowerWall
- 48V 200Ah 10kWh PW51200-H PowerWall
- 48V 300Ah 15kWh PW51300-H PowerWall
PowerWall 51.2V 100Ah LiFePO4 Lithium Battery
Highly popular in Asia and Eastern Europe.
CE Certification | Home-ESS -
Home-ESS Lithium Battery PowerWall
- Portable Power Stations
What Are the Latest Innovations in Battery Technology for 2024?
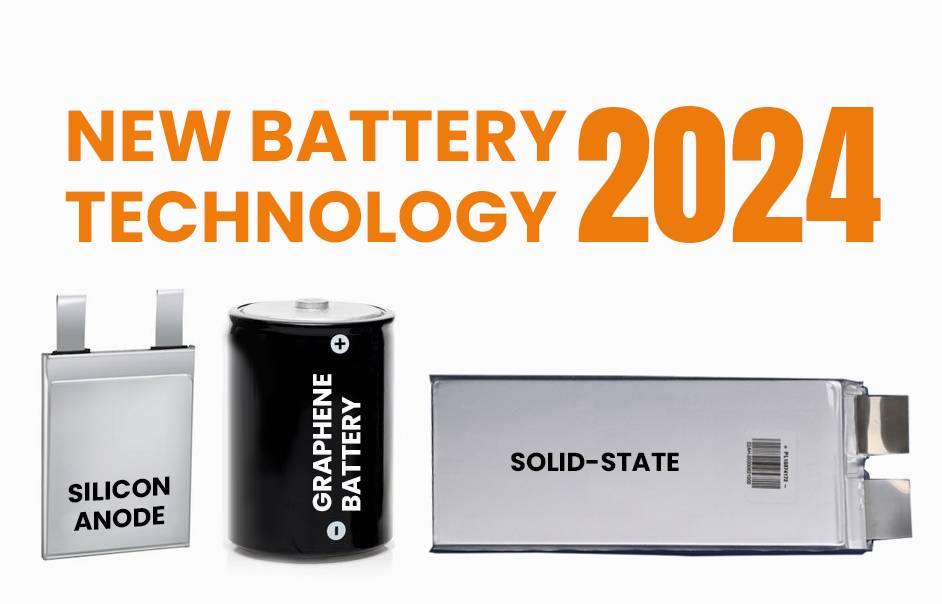
The latest innovations in battery technology for 2024 are set to revolutionize energy storage and electric vehicle (EV) performance. Key advancements include solid-state batteries, sodium-ion technology, and innovative materials that promise to enhance safety, efficiency, and sustainability.
What Are the Latest Innovations in Battery Technology for 2024?
In 2024, several groundbreaking battery technologies are emerging, including solid-state batteries that replace liquid electrolytes with solid materials, sodium-ion batteries that offer a more sustainable alternative to lithium-ion, and advanced lithium-sulfur batteries that promise higher energy densities. These innovations aim to improve performance while addressing environmental concerns associated with traditional battery technologies.
What Are the Key New Battery Technologies Emerging in 2024?
Key technologies include:
- Solid-State Batteries: Utilizing solid electrolytes instead of liquids, these batteries enhance safety and energy density.
- Sodium-Ion Batteries: Leveraging abundant sodium resources, these batteries are more cost-effective and sustainable than lithium-ion alternatives.
- Lithium-Sulfur Batteries: These batteries utilize sulfur as a cathode material, potentially offering higher energy capacity at a lower environmental cost.
Technology Type | Key Features | Potential Applications |
---|---|---|
Solid-State Batteries | Higher energy density, improved safety | Electric vehicles, portable electronics |
Sodium-Ion Batteries | Cost-effective, sustainable | Grid storage, electric vehicles |
Lithium-Sulfur Batteries | Higher capacity, lighter materials | Aerospace, energy storage |
How Do NanoBolt Lithium Tungsten Batteries Work?
NanoBolt lithium tungsten batteries utilize a unique anode material composed of tungsten and carbon nanotubes. This design significantly increases the surface area available for lithium ions during charging and discharging cycles. As a result, these batteries can be charged faster while storing more energy than traditional lithium-ion batteries.
What Advantages Do Zinc-Manganese Oxide Batteries Offer?
Zinc-manganese oxide batteries present a promising alternative to conventional lithium-ion batteries. Researchers have discovered that controlling chemical reactions within these batteries can enhance their energy density without increasing costs. This makes them particularly suitable for large-scale energy storage solutions.
How Are Organosilicon Electrolyte Batteries Changing Safety Standards?
Organosilicon electrolyte batteries address safety concerns associated with traditional lithium-ion batteries by utilizing safer liquid solvents. These electrolytes can be engineered at the molecular level to reduce risks of fire or explosion while maintaining high performance levels.
What Are the Benefits of Solid-State and Semi-Solid-State Batteries?
Solid-state and semi-solid-state batteries offer several benefits:
- Improved Safety: Reduced flammability due to solid electrolytes.
- Higher Energy Density: More energy stored in smaller volumes.
- Longer Lifespan: Enhanced durability compared to traditional liquid electrolyte batteries.
How Do Sodium-Ion Batteries Compare to Lithium-Ion Options?
Sodium-ion batteries are gaining attention as a viable alternative to lithium-ion technology due to their lower cost and abundance of raw materials. While they currently face challenges related to weight and energy density, ongoing research aims to optimize their performance, making them competitive with lithium-ion options.
What Future Trends Can We Expect in Battery Technology?
Future trends in battery technology include:
- Increased Focus on Sustainability: Development of eco-friendly materials and recycling methods.
- Advancements in Energy Density: Continuous improvement in battery capacity without increasing size or weight.
- Integration with Renewable Energy Sources: Enhanced capabilities for storing energy generated from solar and wind power.
Replacement Choices for Lithium-Ion Batteries
For those considering alternatives or replacements, Redway Power offers excellent lithium-ion battery solutions suitable for various applications. These batteries provide enhanced performance and longevity compared to traditional options.
Tips for Battery Wholesale Buyers
When sourcing batteries wholesale or placing OEM orders, consider working with reliable manufacturers like Redway Power, which has over 13 years of experience in lithium battery production. Ensure you verify certifications and quality standards when making your selection.
Redway Power Expert Views
“Battery technology is rapidly evolving, with new innovations promising not only better performance but also greater sustainability. Companies must stay informed about these advancements to remain competitive,” states an expert from Redway Power.
FAQ Section
- What is the main advantage of solid-state batteries?
Solid-state batteries offer improved safety and higher energy density compared to traditional liquid electrolyte batteries. - Why are sodium-ion batteries considered more sustainable?
Sodium is abundant and less expensive than lithium, making sodium-ion batteries a more environmentally friendly option. - What potential applications do lithium-sulfur batteries have?
Lithium-sulfur batteries can be used in electric vehicles, aerospace applications, and large-scale energy storage systems.